CO₂ Storage

What is it?
After CO₂ is captured from industrial processes or directly from air, care must be taken such that this CO₂ does not rapidly enter the atmosphere or otherwise cause harm. While some CO₂ can be beneficially reused, the scale and logistics of CO₂ management dictate that much of the CO₂ that is captured be durably stored, or “sequestered”.
How does it work?
The Earth’s outer layers contain several carbon stocks (also known as “pools” or “reservoirs”). These include the atmosphere (8.3 x 10^2 Gt C, up from 6 x 10^2 Gt C pre-industrial), the ocean (3.8 x 10^5 Gt C), and geologic materials (sediments, crusts and upper mantle, 1 x 10^8 Gt C) (Sundquist and Visser, 2003; Mackenzie and Lerman, 2006; Ciais et al., 2013). The masses of these stocks, listed in parentheses, demonstrate that geologic carbon stocks are by far the largest, followed by oceans, and (last by a long shot) the atmosphere. Combustion of fossil fuels has resulted in a transfer of C from geologic stocks to atmospheric and oceanic stocks. The objective of CO₂ storage is to decrease the buildup of atmospheric CO₂ by shifting the balance towards other stocks, such as oceans or rocks. Here, we focus on geologic CO₂ storage, which is the primary way to store captured CO₂, thereby reversing the carbon fluxes caused by fossil fuel combustion. In addition, geologic CO₂ storage may be complemented by CO₂ storage in other, non-geologic stocks (e.g. increasing ocean alkalinity to store CO₂ (Renforth and Henderson, 2017)).
The most mature technology for CO₂ storage is the injection of CO₂ in deep sedimentary rock formations (Figure 1). Sedimentary rocks are a category of rocks that are formed by deposition of sediment clasts or chemical precipitates and the consolidation (lithification) of these materials into rocks over time. Most prospective sedimentary formations for CO₂ sequestration are located in relatively undeformed areas and consist of vertical successions of porous and impermeable layers. Subsurface pore space of prospective CO₂ storage formations naturally contains either brines (salt water) or hydrocarbons, resulting in saline aquifers or oil and natural gas reservoirs, respectively. For storage, CO₂ is injected in porous layers (reservoir rock) deep underground, while impermeable layers act as a structural trap (cap rock), preventing it from rising back to the surface.
Depths of 800 m or more are targeted for injection because CO₂ exists in supercritical phase at typical temperature and pressure conditions at 800 m depth, thus taking up only 4 % of the volume per unit mass that it would in gaseous phase at Earth surface conditions, with marginal additional density increase per increasing depth (Benson et al., 2005).
CO₂ and brine leakage through a confining layer above the target storage formation is a risk associated with geologic CO₂ storage. Fortunately, CO₂ storage security typically increases over time as primary (structural) trapping mechanisms are replaced by more stable secondary trapping mechanisms. Secondary mechanisms include residual gas trapping (immobilization by capillary forces in the post-injection period), solubility trapping (aqueous dissolution of CO₂), and mineralization through reaction of rock and CO₂-rich fluid (Benson et al., 2005; de Coninck and Benson, 2014; Emami-Meybodi et al., 2015; Krevor et al., 2015; Talman, 2015; Zhang and DePaolo, 2017; National Academies of Sciences, 2019). Of these mechanisms, mineralization is the most permanent because carbonate rocks (such as limestone) are the thermodynamically stable (ground state) form of CO₂ in typical near-Earth-surface conditions and because, once solidified as a carbonate mineral phase, CO₂ is effectively immobile.
In certain other rock types that are rich in alkaline Earth metal - silicate minerals, pilot scale subsurface injections of CO₂ and laboratory-based dissolution/carbonation experiments suggest that large quantities of CO₂ can be mineralized on human timescales (years or less) (Kelemen et al., 2011; Matter et al., 2016). For these reasons, CO₂ mineralization shows promise as a viable and more durable alternative to storage in “conventional” CO₂ storage in sedimentary rocks. Here, we focus on subsurface (in situ) CO₂ mineralization, but rocks can also be mined and carbonated at the surface (ex situ) (Figure 1). Ex situ CO₂ mineralization processes are essentially the same as those that can be applied to alkaline industrial wastes/byproducts, which we discuss separately [link to alkaline industrial byproducts webpage].


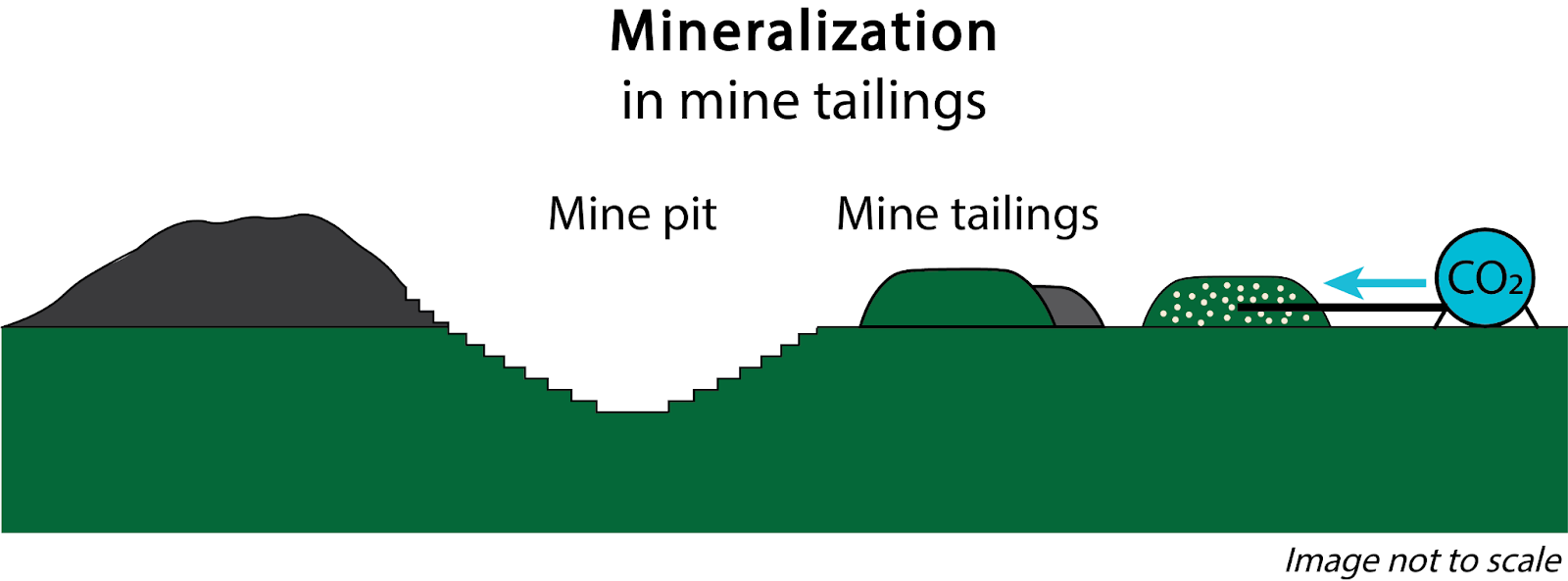

Figure 1. Approaches to CO2 storage in geologic materials.
Why is it important and what are the major barriers?
CO₂ storage is an essential climate change mitigation tool due to its vast capacity. A technical potential of 2,000 Gt CO₂ storage in sedimentary rocks alone is likely this century (Benson et al., 2005). This potential greatly exceeds the quantity of CO₂ that must be captured and stored from fossil fuels and industrial emissions by 2100, which has been estimated at 125 Gt CO₂ (IEA, 2014).
In addition to emissions reductions, CO₂ removal requirements are estimated at 10 Gt CO₂ yr−1 by 2050 and 20 Gt CO₂ yr−1 by 2100 (IPCC, 2018; NASEM, 2019). So-called “conventional” CO₂ utilization pathways—chemicals, fuels, microalgae, building materials and CO₂-enhanced oil recovery—could contribute to between 0.2 Gt CO₂ yr−1 and 3.2 Gt CO₂ yr−1 removed and stored in geologic or biological stocks for centuries or more (Hepburn et al., 2019). The gap between the need to remove CO₂ and the capacity of CO₂ utilization further underscores the importance of CO₂ storage.
Challenges facing the scale up of CO₂ storage include regulations (e.g., slow permitting and uncertain legal liability frameworks), geology (e.g., site characterization), brine handling in response to pore pressure management, as well as real and perceived risks (e.g., induced seismicity, leaks) (NASEM, 2019). Impediments specific to scaling in situ CO₂ mineralization include evolution of reservoir permeability (e.g., clogging with precipitates vs. reaction-driven cracking), and injection approaches that use large volumes of water, which could contribute to use of seawater and offshore drilling, which may come with increased costs (Kelemen and Matter, 2008; Matter et al., 2016; NASEM, 2019; Tutolo et al., 2021).
What we do
Contributions in our group to the field of CO₂ storage include technological and policy assessment and outlook (Kelemen et al., 2019), mapping and geospatial analysis (Pilorgé et al., 2021), and energetic, emissions, and cost estimation associated with compression, transport, and injection of CO₂ (NASEM, 2019). Ongoing work includes systems engineering at regional levels with an emphasis on transport modeling. Further, questions surrounding the handling of brines that may be produced for pore pressure management is a developing research area among our team.
Additional reading and references:
Benson S., Cook P., Anderson J., Bachu S., Nimir H. B., Basu B., Bradshaw J., Deguchi G., Gale J., von Goerne G., Heidug W., Holloway S., Kamal R., Keith D., Lloyd P., Rocha P., Senior B., Thomson J., Torp T., Wildenborg T., Wilson M., Zarlenga F., Zhou D., Celia M., Gunter B., King J. E., Lindeberg E., Lombardi S., Oldenburg C., Pruess K., Rigg A., Stevens S., Wilson E., Whittaker S., Borm G., Hawkins D. and Lee A. (2005) Underground geological storage. In IPCC Special Report on Carbon Dioxide Capture and Storage (eds. B. Metz, O. Davidson, H. DeConinck, M. Loos, and L. Meyer). Cambridge Univ Press, Cambridge, UK. pp. 195–276.
Ciais P., Sabine C., Bala G., Bopp L., Brovkin V., Canadell J., Chhabra A., DeFries R., Galloway J., Heimann M., Jones C., Le Quéré C., Myneni R. B., Piao S. and Thornton P. (2013) Carbon and Other Biogeochemical Cycles. In Climate Change 2013: The Physical Science Basis. Contribution of Working Group I to the Fifth Assessment Report of the Intergovernmental Panel on Climate Change (eds. T. F. Stocker, D. Qin, G.-K. Plattner, M. Tignor, S. K. Allen, J. Boschung, Y. Xia, V. Bex, and P. M. Midgley). Cambridge Univ Press, Cambridge, United Kingdom and New York, NY, USA.
de Coninck H. and Benson S. M. (2014) Carbon Dioxide Capture and Storage: Issues and Prospects. Annu. Rev. Environ. Resour. 39, 243–270.
Emami-Meybodi H., Hassanzadeh H., Green C. P. and Ennis-King J. (2015) Convective dissolution of CO2 in saline aquifers: Progress in modeling and experiments. Int. J. Greenh. Gas Control 40, 238–266.
Hepburn C., Adlen E., Beddington J., Carter E. A., Fuss S., Mac Dowell N., Minx J. C., Smith P. and Williams C. K. (2019) The technological and economic prospects for CO 2 utilization and removal. Nature 575, 87–97.
IEA (International Energy Agency) (2014) World Energy Outlook 2014.,
IPCC (2018) Global warming of 1.5°C. An IPCC Special Report on the impacts of global warming of 1.5°C above pre-industrial levels and related global greenhouse gas emission pathways, in the context of strengthening the global response to the threat of climate change, sustainable development, and efforts to eradicate poverty.,
Kelemen P. B. and Matter J. (2008) In situ carbonation of peridotite for CO2 storage. Proc. Natl. Acad. Sci. 105, 17295–17300.
Kelemen P. B., Matter J., Streit E. E., Rudge J. F., Curry W. B. and Blusztajn J. (2011) Rates and Mechanisms of Mineral Carbonation in Peridotite: Natural Processes and Recipes for Enhanced, in situ CO2 Capture and Storage. In Annual Review of Earth and Planetary Sciences, Vol 39 (eds. R. Jeanloz and K. H. Freeman). Annual Reviews, Palo Alto. pp. 545–576.
Kelemen P., Benson S. M., Pilorgé H., Psarras P. and Wilcox J. (2019) An Overview of the Status and Challenges of CO2 Storage in Minerals and Geological Formations. Front. Clim. 1.
Krevor S., Blunt M. J., Benson S. M., Pentland C. H., Reynolds C., Al-Menhali A. and Niu B. (2015) Capillary trapping for geologic carbon dioxide storage - From pore scale physics to field scale implications. Int. J. Greenh. Gas Control 40, 221–237.
Mackenzie F. T. and Lerman A. (2006) Brief Overview of Carbon on Earth. In Carbon in the Geobiosphere — Earth’s Outer Shell — (eds. F. T. Mackenzie and A. Lerman). TOPICS IN GEOBIOLOGY. Springer Netherlands, Dordrecht. pp. 1–22.
Matter J. M., Stute M., Snæbjörnsdottir S. Ó., Oelkers E. H., Gislason S. R., Aradottir E. S., Sigfusson B., Gunnarsson I., Sigurdardottir H., Gunnlaugsson E., Axelsson G., Alfredsson H. A., Wolff-Boenisch D., Mesfin K., Taya D. F. de la R., Hall J., Dideriksen K. and Broecker W. S. (2016) Rapid carbon mineralization for permanent disposal of anthropogenic carbon dioxide emissions. Science 352, 1312–1314.
NASEM (2019) Negative Emissions Technologies and Reliable Sequestration: A Research Agenda., The National Academies Press, Washington, DC.
National Academies of Sciences E. (2019) Negative Emissions Technologies and Reliable Sequestration: A Research Agenda.,
Pilorgé H., Kolosz B., Wu G. C. and Freeman J. (2021) Global Mapping of CDR Opportunities. In CDR Primer (eds. J. Wilcox, B. Kolosz, and J. Freeman).
Renforth P. and Henderson G. (2017) Assessing ocean alkalinity for carbon sequestration. Rev. Geophys. 55, 636–674.
Sundquist E. T. and Visser K. (2003) The Geologic History of the Carbon Cycle. In Treatise on Geochemistry (eds. H. D. Holland and K. K. Turekian). Pergamon, Oxford. pp. 425–472.
Talman S. (2015) Subsurface geochemical fate and effects of impurities contained in a CO2 stream injected into a deep saline aquifer: What is known. Int. J. Greenh. Gas Control 40, 267–291.
Tutolo B. M., Awolayo A. and Brown C. (2021) Alkalinity Generation Constraints on Basalt Carbonation for Carbon Dioxide Removal at the Gigaton-per-Year Scale. Environ. Sci. Technol. 55, 11906–11915.
Zhang S. and DePaolo D. J. (2017) Rates of CO2 Mineralization in Geological Carbon Storage. Acc. Chem. Res. 50, 2075–2084.