Direct Air Capture
Most experts agree that we'll need to remove CO2 from the atmosphere to limit catastrophic effects from climate change. One approach you’re going to hear a lot about is Direct Air Capture, or DAC. This brief overview is designed to introduce you to DAC: what it is, how it works, and why it's so important in our fight against climate change.
What is Direct Air Capture?
Direct Air Capture is a technology that removes carbon dioxide (CO2) directly from the air using engineered machines. There are many ways to remove CO2 from the atmosphere. In fact, the Earth does this already through natural “ sinks” in the ocean and biosphere (e.g., trees and soils). DAC seeks to add to this natural carbon removal to help reduce atmospheric accumulation of CO2. There are few things that set apart DAC from other solutions, both good and bad. Before we dive into the costs and benefits, let's first explore how it works.
How Does DAC Work?
In Step 1, ambient air flows through building-like structures where filters react with and trap CO2. These structures are often referred to as air contactors, because it is the place where air makes contact with the filter. The internal structure is optimized for maximum air contact and is made up of chemistry that selectively binds to CO2, while allowing the other components of air (predominantly N2 and O2) to pass through and exit back to the atmosphere. In Step 2, CO2 is released from the filter in a controlled way, usually by applying heat or some type of secondary reaction.
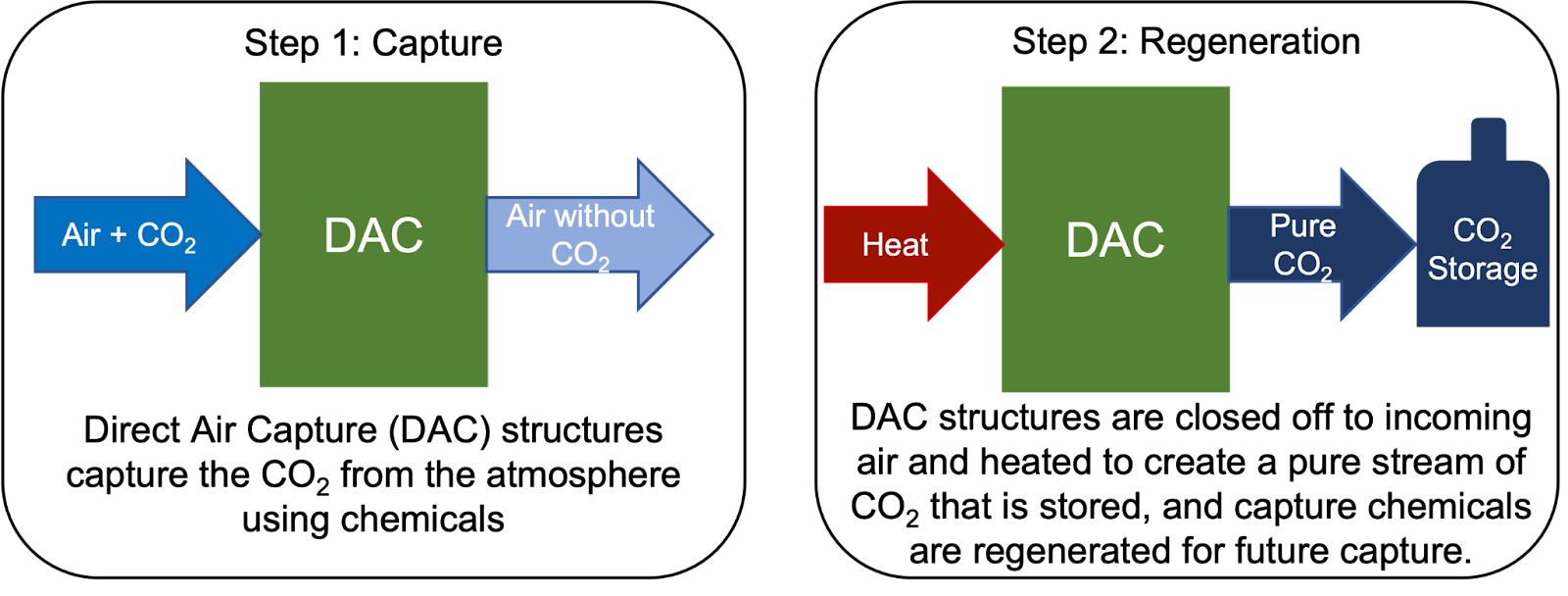
There are two primary methods for DAC. One uses solid sorbent material that is selectively reactive with CO2 in the atmosphere. In solid sorbent systems, the air is pushed through solid sorbent material, where the CO2 becomes bound, allowing the other components of air to flow through. Once the sorbent material is saturated with CO2, the material is isolated from the air, the pressure is lowered, and the temperature is elevated. This allows the CO2 to release from the sorbent material and be collected for further compression and storage. One company that is pursuing this approach is Climeworks.

Another method uses liquid solvents to extract the CO2 from air. Instead of passing over a fixed, solid filter, the air passes through a “shower” of liquid solvent where it reacts with a chemical dissolved in the water (like potassium hydroxide (KOH)), which extracts the CO2 out of the air stream. To regenerate the capture liquid in this process, a series of chemical reactions are performed, using calcium hydroxide (Ca(OH)2) to form calcium carbonate (CaCO3) and looping that through a high-temperature step, isolating the CO2 for transportation and storage. One company that is pursuing the liquid solvent method is Carbon Engineering.
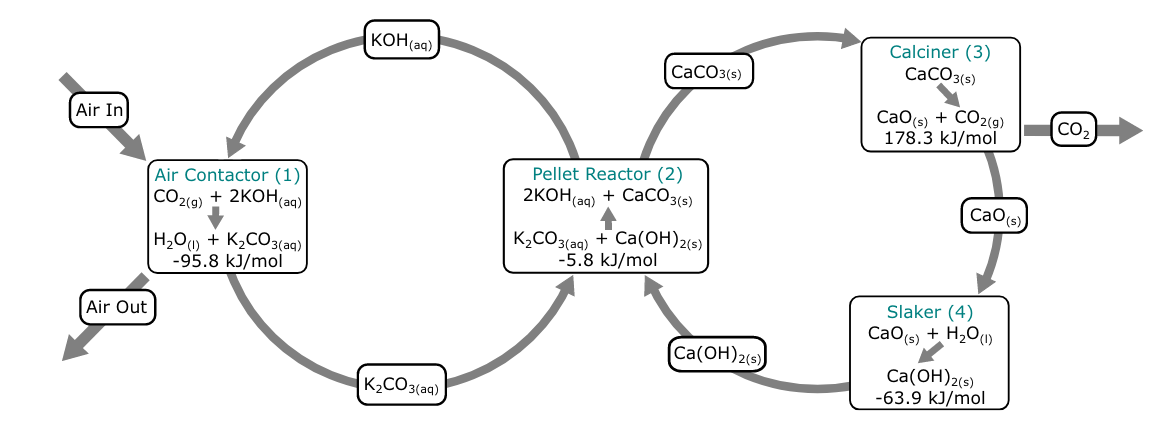
Recently, further investigation into using naturally occurring minerals to uptake CO2 directly from the atmosphere has also been conducted. One company pursuing this method is Heirloom Carbon Technologies, which was co-founded by a former CEC Lab member, Dr. Noah McQueen. The Heirloom DAC process begins first with calcium carbonate material (CaCO3), which is then calcined, forming calcium oxide (CaO; or lime) and CO2. The CO2 in this step is captured for transportation and storage. The resulting CaO is hydrated, forming calcium hydroxide (Ca(OH)2), and then laid out in a contactor structure, where it will ambiently carbonate, forming CaCO3 again. The CaCO3 is then cycled through the calciner again, capturing the CO2 for transportation and storage
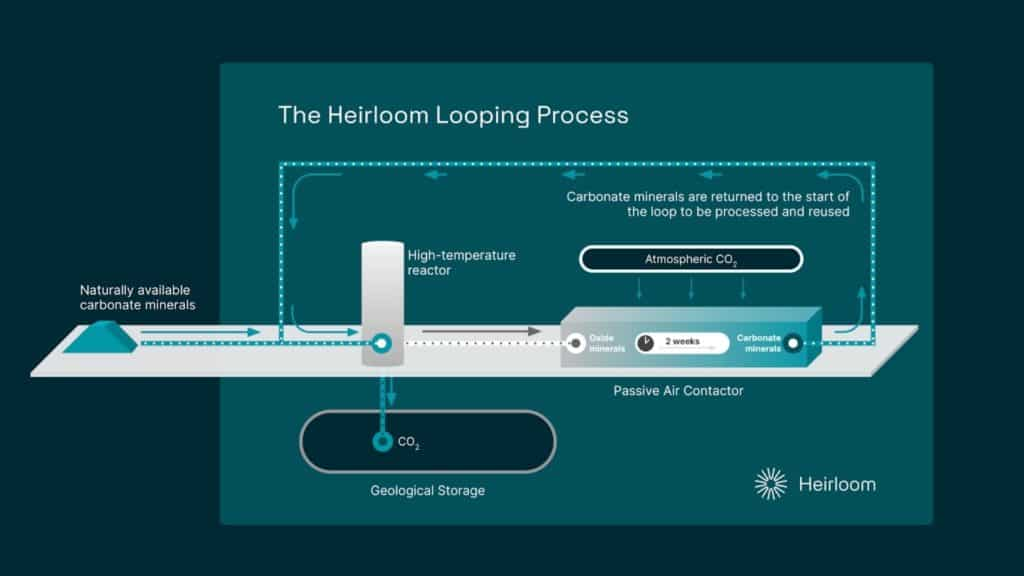
These are not the only methods for achieving DAC. There are many others, including electrochemical DAC being pursued by Verdox and humidity-swing adsorption being pursued by Silicon Kingdom Holdings and ASU, in a capture device called Mechanical Trees, but these are some of the ones that are more extensively documented in the academic literature.
Why is it Important and What Are the Major Barriers?
DAC is one of the few engineered solutions to removing CO2 directly from the atmosphere. A notable feature of DAC is that it is not dependent on biological processes, unlike some carbon dioxide removal (CDR) methods, and as such does not rely on arable land for deployment. This will be crucial when considering how CDR solutions pose the potential to compete with food production at large scales. Additionally, the ability for DAC to be sited nearly anywhere in the world can also be advantageous when minimizing the distance between capture and storage sites.
However, there are still barriers to implementation and many ways to contribute to DAC’s commercialization. One of the major barriers is the large energy requirements for removing CO2 from the atmosphere. In order to remove one million tonnes of CO2 from the atmosphere, it will require 300-500 MW, the same amount of energy that can be produced from a power plant. These high energy requirements can also result in higher costs for deployment. In the absence of a carbon price (price of emitting CO2) or adequate compensation for CO2 removal from the atmosphere, it can be difficult to finance DAC projects. These costs may be made up through the ability to sell CO2 as a product, but depending on how the CO2 is then utilized or stored, will depend on if it will return to the atmosphere.
Another potential barrier for the scale up of DAC could be the limited existing options for permanent CO2 storage (link to storage page). It has been proposed to store CO2 in geologic formations where it would be secure for millenia, however, in order to do this Class VI wells are needed for injection. The extensive permitting process and regulations surrounding these wells may be prohibitive in deploying secure storage for large DAC processes in the near-term.
It should also be noted that DAC is just the method to remove the CO2 from the atmosphere, the true removal will depend on the life cycle of CO2 and how the CO2 is then either utilized or stored.
What Does the CEC Lab Do?
In the Clean Energy Conversions Lab (CECL), we analyze and assess leading DAC technologies through techno-economic assessments, life cycle analyses, mapping/siting studies and from an environmental justice perspective. We have furthered the field by completing techno-economic assessments for various DAC approaches powered by multiple different energy sources and using mapping strategies to illustrate places where DAC may be best deployed for access to low-carbon energy and CO2 storage.
Additional Reading and Resources
- A New Way to Remove CO2 from the Atmosphere (2018)
- Carbon Dioxide Removal (CDR) Primer (2021)
- Direct Air Capture: Resource Considerations and Costs for Carbon Removal (2021)
- A Review of Direct Air Capture (DAC): Scaling Up Commercial Technologies and Innovating for the Future (2021)
- Natural Gas vs. Electricity for Solvent-Based Direct Air Capture (2021)
- Cost Analysis of Direct Air Capture and Sequestration Coupled to Low-Carbon Thermal Energy in the United States (2020)
- Faradaic Electro-Swing Reactive Adsorption for CO2 Capture (2019)
- A Scalable Direct Air Capture Process Based on Accelerated Weathering of Calcium Hydroxide (2022)